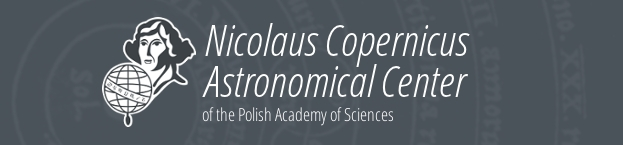
Samuel Lander (CAMK, Warsaw)
Neutron stars host the strongest known magnetic fields in the Universe. These fields play several key roles in the evolution and dynamics of neutron stars, as well as powering the emission which allows us to observe them. Unlike normal stars, like the Sun, neutron stars have no mechanism for continuously regenerating their magnetic fields, so the fields which exist must be in a dynamically stable equilibrium in order to survive. Since we have no direct probe of the interior magnetic field of neutron stars, we must resort to theoretical models. Realistic models need, however, to incorporate a challenging piece of neutron-star physics: the fact that the star’s core forms a superconducting fluid. We describe how to account for the microscopic physics of superconductivity in large-scale models of the whole neutron star, and present some of the first equilibrium models for magnetic fields in the star's superconducting core. We conclude by looking at the interplay between rotation and magnetic fields in neutron stars, and show how the star’s rotation and magnetic axes can evolve to become either aligned or orthogonal.
Michela Mapelli (University of Padova)
On September 14 2015, the LIGO interferometers captured a gravitational wave (GW) signal from two merging black holes (BHs), opening the era of GW astrophysics. Five BH mergers have been reported so far, three of them involving massive BHs (>30 solar masses). According to stellar evolution models, such massive BHs can originate from massive relatively metal-poor stars. Understanding the formation of binary BH systems (BHBs) is a major challenge for astrophysics: it involves a plethora of dynamical and stellar-evolution processes, which can be studied only via advanced numerical codes. In this talk, I will discuss the main formation pathways of merging BHs with particular attention to the main open questions and to the next frontiers of population synthesis and dynamical models.
Rainer Spurzem (National Astronomical Observatories of China)
Black Holes are difficult to detect through electromagnetic radiation alone. Indirect evidence for their existence in our universe has been accumulated on a large range of black hole mass scales (supermassive in galactic nuclei, stellar in X-ray binaries and most notably recently by direct gravitational wave detection through LIGO/Virgo). Intermediate mass black holes may exist too, but remain the most speculative variety of their kind. Recently, gravitational waves from coalescing stellar mass black holes have been detected by the LIGO instruments. This is considered a first direct evidence of the existence of black holes and their binaries. In our team we have been working on the interaction of black holes on all mass scales with stellar clusters surrounding them - globular and nuclear star clusters with stellar mass black holes and galactic nuclei with supermassive ones. We study with computer simulations based on direct N-body simulations with Post-Newtonian general relativity (if needed) how black holes form, build binaries, evolve, disrupt stars, and emit gravitational waves. Recently in collaboration with CAMK we are exploring ways to form intermediate mass black holes. The talk will give an overview of simulations, methods used and results obtained. Astrophysical topics are for example the ``detection'' of black hole mergers like observed by LIGO in our N-body models, and the merger of supermassive binary black holes in galactic nuclei, with prediction of expected low-frequency gravitational wave emission.
Nicolas Chamel (Université Libre de Bruxelles)
Formed in the aftermath of gravitational core-collapse supernova explosions, neutron stars are the most compact observed stars. Their average density exceeds than that found inside the heaviest atomic nuclei. Neutron stars are also endowed with the strongest magnetic fields known, which can reach millions of billions times that of the Earth. According to our current understanding, a neutron star is stratified into distinct layers. The surface is probably covered by a metallic ocean. The solid layers beneath consist of a crystal lattice of pressure-ionized atoms embedded in a highly degenerate electron gas. With increasing density, nuclei become progressively more neutron rich until neutrons start to drip out of nuclei thus delimiting the boundary between the outer and inner regions of the crust, where neutron-proton clusters are immersed in a neutron liquid. At about half the density of heavy nuclei, the crust dissolves into an homogeneous liquid mixture of nucleons and leptons. The composition of the innermost part of the core remains highly uncertain. During this talk, our developments of unified and thermodynamically consistent equations of state of dense matter in neutron stars will be reviewed.